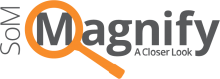
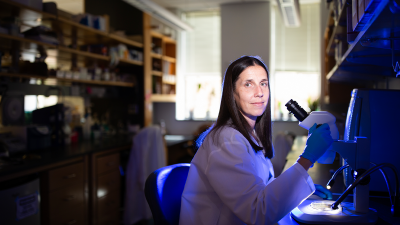
The Power and Promise of RNA
The word messenger RNA, or mRNA for short, went from scientific jargon to everyday conversation during the pandemic because of the molecule's starring role in COVID-19 vaccines.
Messenger RNA contains blueprints for proteins that do all sorts of jobs in our bodies. In COVID-19 vaccines, it contains instructions to make proteins similar to the spikes on the coronavirus. This encourages the immune system to create antibodies to fight the virus if we encounter it in the future.
Vaccines are just the beginning of the therapeutic potential of RNA. Scientists at Duke University School of Medicine have long probed the mysteries of RNA, with an eye on harnessing its power for new and better therapies for cancer, diabetes, heart disease and more.
RNA translates our genetic code into action, using information in our genes to create a functioning organism. But the body has ways to modify RNA to change gene expression. These RNA modifications, crucial for normal development, sometimes go awry in disease states.
At Duke, several scientists study RNA modifications. “That’s an area of strength for us,” said cardiologist Christopher Holley, MD, PhD, associate professor of medicine and assistant research professor in Department of Molecular Genetics and Microbiology. “We can’t think of any other university in the world that has as large a group studying RNA modifications as there is here at Duke.”
Manipulating RNA Modifications
Unlike mRNA, not all RNA contains blueprints for proteins. Some types of RNA guide the modification of mRNA, essentially turning genes on or off without changing the genetic code itself.
In RNA, the genetic code is written in base chemicals referred to by the letters A, G, C, and U. Holley compares RNA modifications to an asterisk. “These modifications don’t change the letter sequence of RNA,” he said. “You still have the same ‘word,’ but there is some extra information.”
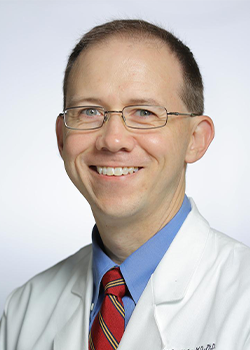
Holley studies a type of modification-guiding RNA called small nucleolar RNA, or snoRNA. While snoRNAs have a role in normal biology, they are also active in some unhealthy processes, including oxidative stress, which damages cells.
Holley has found that turning snoRNAs off in mice protects the mice from diabetes, atherosclerosis, and the symptoms of sickle cell disease, with no apparent side effects. “There seems to be a beneficial effect of dialing down these snoRNAs,” he said, “because we think they really promote oxidative stress damage.”
Holley, whose doctorate is in pharmacology, is designing a molecule that will attach itself to snoRNAs, causing them to self-destruct. With the help of Duke’s Office for Translation & Commercialization, Holley and Brittany Elliott, PhD, senior research scientist, launched a company called snoPanther to help bring the idea to market.
“The dream is that we would be able to turn these into drugs for people,” he said.
He’s especially interested in developing better treatments to help his patients avoid heart attacks. He’s actively pursuing snoRNA treatments for diabetes and sickle cell disease as well.
“There are hundreds of snoRNAs,” he said, “and we think in general it could be a whole new class of molecules we could target for drug development.”
Mapping RNA Modifications
One of the reasons Kate Meyer, PhD, assistant professor in biochemistry, came to Duke was the concentration of RNA researchers here. “That was a big draw for me,” Meyer said. “It’s great because we all study similar concepts but we’re different enough that we don’t compete with each other; we complement each other.”
Meyer studies a modification called m6A, in which a molecule called a methyl group gets added to a particular site on RNA. “Proper regulation of m6A is required for cells and organisms to function and for animals to develop normally,” she said. “Dysregulation of m6A has been linked to a variety of different diseases, most notably several cancers.”
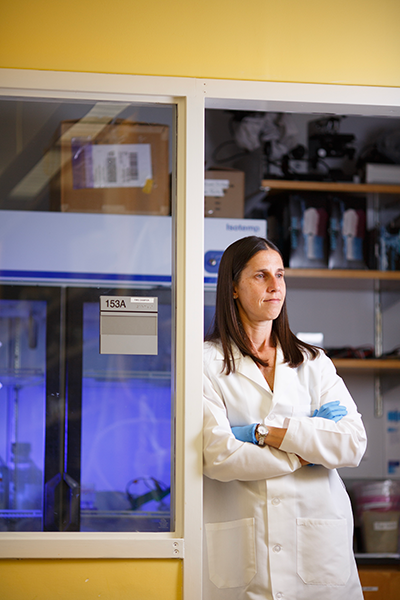
When she was a postdoctoral researcher, Meyer helped develop the first technique to map m6A sites in cells. At Duke, she and her lab members have developed new methods which can detect m6A from very low amounts of RNA, allowing researchers to zoom in and identify sites in a single cell.
“Single-cell m6A profiling has provided new insights into m6A biology,” she said. The new technique revealed about 170,000 m6A sites throughout the body – many more than scientists had imagined.
Meyer, who is particularly interested in neuroscience applications, studies the functions of m6A in the brain, where m6A is known to be active in response to axonal injury, neural diseases, and brain cancer.
“The more we understand about methylation and how it is regulated in cells,” she said, “the better positioned we are to develop novel therapeutics.”
Meyer recently served on the National Academies of Sciences, Engineering and Medicine committee that compiled a report providing a roadmap for achieving the complete sequencing of RNA molecules and their modifications from one end to the other. Meyer believes this feat will help enable researchers, clinicians, and the biotechnology sector to more fully harness the power of RNA.
Recognizing RNA
Before Meyer joined the Duke faculty, Stacy Horner, PhD, associate professor in integrative immunobiology, came across Meyer’s postdoctoral paper mapping m6A sites. Horner decided to use the technique in her own lab, in a slightly different application.
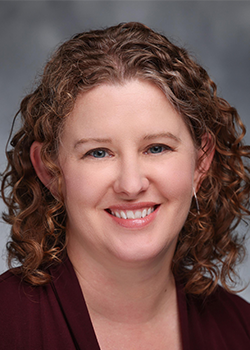
Horner studies RNA in viruses and she wanted to look for m6A in viral RNA. “I felt like we should look at this because no one was exploring this,” she said, “and then, with her work, we were able to do this.”
She found that viral RNA, like our RNA, does contain m6A sites, a finding that is informing further research. “We have been looking at how proteins in the body might sense a specific pattern in viral RNA that contains these modifications,” she said.
Her overall goal is to understand how our bodies distinguish viral RNA (which the immune system should attack) from our own RNA (which the immune system should ignore).
In illuminating these biological mechanisms, Horner’s research could lead to treatments for autoimmune diseases in which the body's immune system attack its own RNA. “You need to know the biochemical mechanisms that distinguish viral RNA from our own RNA so you know what to target,” she said.
Her work will also be important in understanding how to design RNA therapeutics so that the body doesn’t identify them as something to attack.
Horner, who also has appointments in the departments of cell biology, medicine, molecular genetics and microbiology, and the Duke Cancer Institute, now works alongside Meyer to co-direct the Center for RNA Biology, the intellectual home for RNA research at Duke.
“We share what we’re learning and we share technology,” she said. “It really helps us push the envelope.”
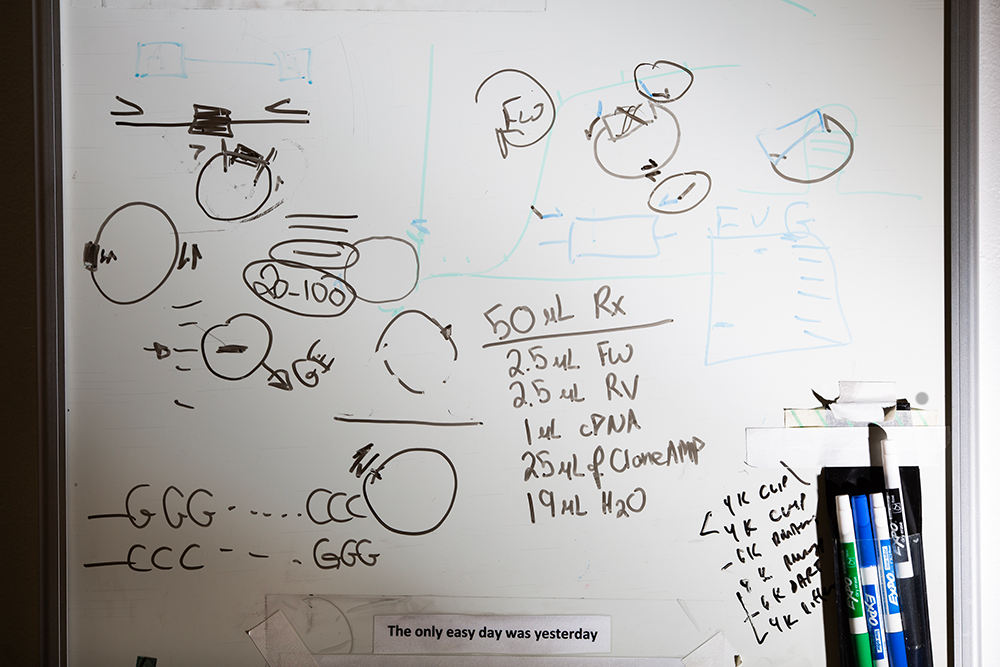
Programming RNA
As a relative newcomer to RNA research, Josh Huang, PhD, the Duke School of Medicine Distinguished Professor of Neuroscience, appreciates the “rich environment” of Duke’s in-house expertise, which has helped him get up to speed on RNA after years of studying neural circuitry.
He’s interested in using RNA as a tool to learn more about cell types and to manipulate cells to treat disease.
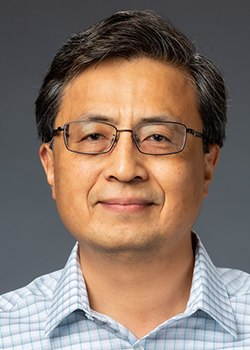
He has recently developed a technique he calls CellREADR to “program” engineered mRNA to bind to RNA in particular cells in the body and deliver instructions.
Imagine the target sequence is in RNA in a cancer cell. Once the engineered mRNA is attached to the cancer RNA, it issues instructions. “It’s a message that we want to deliver to the cancer cell,” Huang said, “to tell the cancer cell to die or to label the cancer cell so that immune cells will kill it.”
READ MORE New RNA-based tool can illuminate brain circuits, edit specific cells
The technology has applications far beyond cancer. In Parkinson’s disease patients, for example, engineered mRNA could locate cells involved in synthesizing dopamine, attach to the RNA in those cells, and deliver instructions to fix the malfunction.
Like Holley, Huang has started a company to bring his technology to market, called Doppler Bio, with help from Duke’s Office for Translation & Commercialization.
The Promise of RNA
RNA therapies are quicker and less expensive to manufacture than more traditional pharmaceuticals, which is one of the reasons the COVID-19 vaccines were designed and produced so quickly. They also have the potential to be easily tailored for different vaccines and disease treatments.
One of the most exciting benefits for patients is the possibility of increased effectiveness with fewer side effects.
In the case of cancer, say, RNA therapy could potentially destroy cancer cells without affecting other cells. This contrasts with currently available radiation and chemotherapy, which damage a broad swath of normal cells in the body.
“Broadly speaking, that is the promise of RNA therapeutics – precision and effectiveness,” Huang said.
Mary-Russell Roberson is a freelance writer in Durham.
Eamon Queeney is assistant director of multimedia and creative at the Duke School of Medicine.