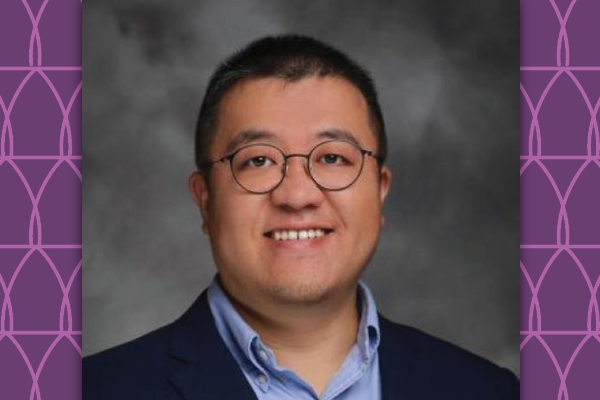
Muscle injuries are one of the most common types of injuries: from sprains and strains to bruises and lacerations. Our bodies, though, have the remarkable ability to heal from these injuries, thanks to muscle stem cells, which take up residency in our muscles and mostly stay dormant until injury. In the face of an injury, some of the cells spring into action and mature into functional cells to repair the damage, while the remaining muscle stem cells remain immature and replenish the stem cell supply.
But how do these cells determine who stays and who goes?
Yarui Diao, PhD, assistant professor of cell biology, and team provided the first temporally resolved single-cell chromatin accessibility atlas of skeletal muscle regeneration and were able to determine what makes some muscle stem cells differentiate into other cell types while others stay as stem cells. Their results, published in Journal of Cell Biology, could translate into new therapies for muscular disorders.
“We know that muscle stem cells don’t become depleted after multiple injuries,” said Arinze Okafor, co-first author and a fourth-year graduate student in the Diao lab. “There has to be a way that some of the cells know not to differentiate and instead expand and reoccupy the original stem cell niches, so that even after multiple rounds of injury, the stem cell pool regenerates instead of depletes.”
Studying this is tricky, though, because once you take muscle stem cells out of the body, they tend to spontaneously differentiate, making it challenging to explore their self-renewal properties. Once a stem cell has committed to differentiating, it loses its stem cell potency, meaning it can’t repopulate the stem cell pool.
Using frozen muscle tissue from mice, the Diao lab employed single-nuclei ATAC-seq to capture the chromatin profiles of muscle stem cells at various stages of regeneration, from pre-injury to 6 and 12 hours after injury, up to several days after injury. “This provided us with high resolution data, higher than what has been done in previous studies in the past,” Okafor said. “It allowed us to identify the different trajectories these cells take after injury.”
They created an accessibility atlas that provides a detailed look at the gene regulation of muscle regeneration in vivo.
They discovered that the cell membrane protein marker Betaglycan indicates the cells that remain stem cells. Muscle cells high in Betaglycan protein remain stem cells, while those without it differentiate. “This allows us to isolate, transplant, and contribute to skeletal muscle regeneration,” said Dr. Xin Lin, another co-first author of the study, “which provides a new cellular framework for future studies of muscle regeneration and stem cell self-renewal in general.”
Knowing how to maintain stem cells outside of an organism will allow for more experimentation, which could lead to more avenues to create therapeutics for muscle-based diseases, such as Duchenne muscular dystrophy. “If you have certain mutations in the dystrophin gene, then you have this disease,” Diao said. “With an improved control of stem cell self-renewal, scientist and doctors could correct such genetic mutations in the stem cells themselves, rather than in the mature muscle, and put the stem cells back in the body to correct the disease.”
Due to their retained stemness, these muscle stem cells, once re-inserted into the patient, ideally would form new healthy fibers and populate other stem cell niches, and effectively phase out the disease, although a substantial amount of work still needs to be done to get there.
For now, Okafor and Lin are just excited to put out this first-of-its-kind resource for the scientific community. “It’s exciting to be involved in solving a decades-long mystery of how muscle stem cells don’t deplete when we undergo regeneration after injury,” Okafor said.